Introduction to Tribology
What is Tribology?
Tribology is the study of surfaces moving relative to one another, a phenomenon that affects our lives in a multitude of ways every day. The term tribology is based on the Greek word for rubbing and, although the term itself was not coined until 1964, there are images of tribology in action from as long ago as ancient Egypt, when early tribologists used oil to help facilitate sliding of large statues. Generally, tribology includes three key topics: friction, wear and lubrication. Friction is the resistance to relative motion, wear is the loss of material due to that motion, and lubrication is the use of a fluid (or in some cases a solid) to minimize friction and wear. The field is necessarily interdisciplinary and utilizes skills from mechanical engineering, materials science and engineering, chemistry and chemical engineering and more. Tribology is both technologically-relevant and scientifically-fascinating, and it's definitely an exciting time to be a tribologist!
To see a short video about tribology and our research group, click here.
Why is Tribology Important?
Traditional Applications
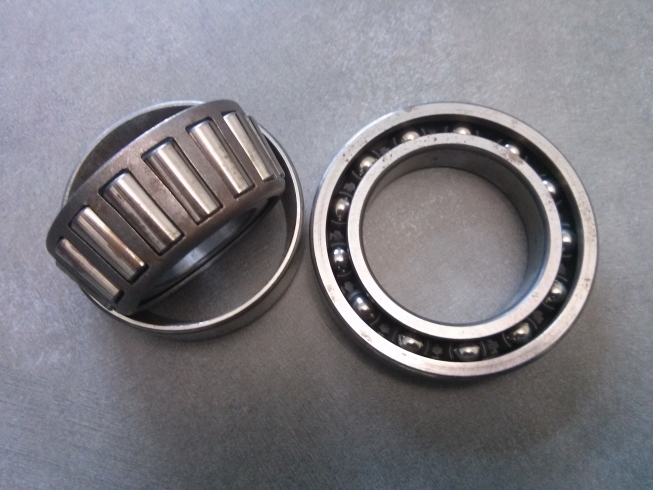
≪ More about traditional applications of tribology ≫
Most mechanical components have one or more moving parts.
This means that something is moving relative to something else, so there is tribology happening.
In some components, such as bearings and gears, the goal is to minimize the resistance to sliding or rolling so that as little energy as possible is lost to friction.
In other components, such as brakes and clutches, we want to maximum sliding resistance in order to limit the relative motion.
There are also many manufacturing processes that rely on tribology, such as rolling, turning, stamping, grinding and polishing. Further, most transportation methods depend on tribology, not only within the mechanical components that drive them, but also at the contact between the wheels and the surfaces on which they slide or roll. There are also examples of tribology in construction and exploration equipment such as excavators, oil rigs, mine slurry pumps and tunnel digging drills. The processes of friction and wear, and the use of lubricants to control friction and wear are ubiquitous in a variety of industries.
|
Everyday Examples of Tribology

≪ More applications of tribology in your everyday life ≫
There are many examples of tribology and tribology-enabled function in the world of sports.
For example, the bottoms of athletic shoes are fine-tuned to provide just the right amount of resistance to sliding for a given sport.
Also, footballs and balls for other sports have to be designed to be grip-able, but not too sticky.
There are many examples in sports equipment where tribology can be the difference between winning or not.
Common examples in winter sports include snow skis, bobsleds and curling stones.
There are also many natural processes where tribology is relevant. Some of these processes occur on very large length scales. For example, earthquakes occur when friction builds up over time until the earth cannot withstand the force and there is a shift, and erosion due to water or wind is the process of the earth wearing away over time. Other tribological phenomena occur on much smaller scales. For example, the feet of geckos have evolved to enable them to preferentially stick to surfaces (or not) so that they can effectively walk on walls. Also, scales on snakes provide the specialized contact with the ground that they need to move along both quickly and quietly. Nature has found many innovative solutions to tribological challenges. Lastly, there are many other examples of tribology in fields as varied as music - for example drawing a bow across violin strings to play notes - and cosmetics - where significant resources are invested to make skin or hair products that have the right feel. Pretty much everywhere we turn there is another example of tribology!
|
Role of Tribology in Energy Efficiency

≪ More about energy wasted due to friction and wear ≫
Global energy consumption is expected to grow in upcoming years, straining both resources and the environment.
At the same time, a huge amount of energy is lost to friction: for example, seven quads of energy are wasted annually due to friction in passenger cars globally1.
Further waste occurs due to wear of contacting materials, as the energy required to replace parts is substantial2, and the economic, environmental, and safety costs of wear-induced failures can be extensive3.
Moreover, many of the challenges facing new energy-efficient technologies - such as wind turbines - are tribological in nature4.
Therefore, tribology is critically important to addressing some of the world's key issues related to energy efficiency and the economic and societal implications of energy usage.
![]() [1] Holmberg, K., Andersson, P. and Erdemir, A. Global Energy Consumption Due to Friction in Passenger Cars. Tribology International 47, 221-234 (2012). [2] EIA. 1994 Manufacturing Energy Consumption Survey (MECS). Energy Information Administration, U.S. Department of Energy, Washington, DC. (1997). [3] Jost, H.P. Tribology Micro Macro Economics: A Road to Economic Savings. Tribology and Lubrication Technology 61, 18-22 (2005). [4] Sheng, S., Oyague, F. and Butterfield, C.P. Investigation of Various Wind Turbine Drive Train Condition Monitoring Techniques. (National Renewable Energy Laboratory, 2010). |
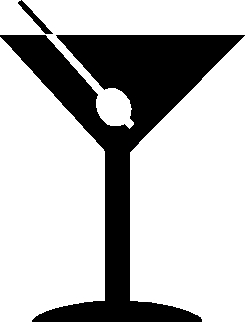
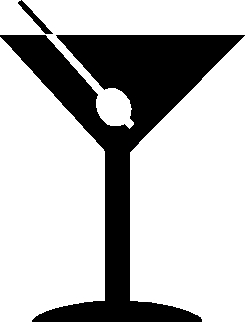
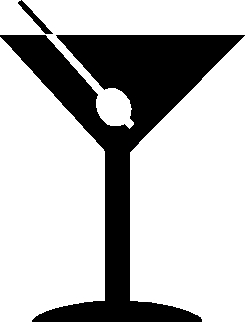
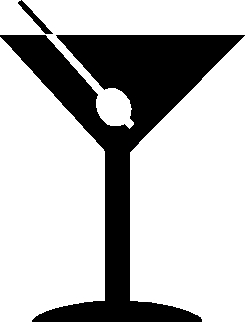
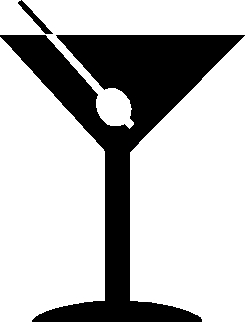
Tribology 101
Tribology is an interdisciplinary field that includes mechanical engineering; materials science and engineering; chemistry and chemical engineering; and more. This wide variety of skills is necessary because many different physical phenomena occur at a sliding interface. There are also many different areas of focus within tribology. Generally speaking, there are three major topics within tribology: friction, wear and lubrication. Each of these is described in more detail below.Friction
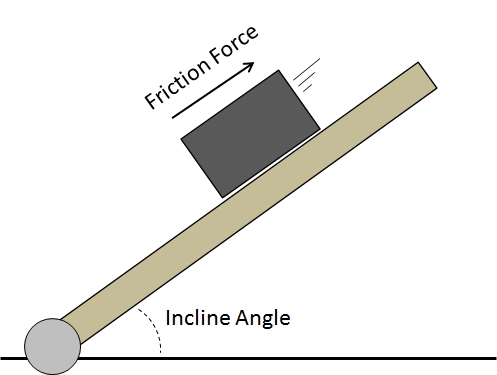
≪ More about friction ≫
Friction is the tangential resistance to motion between two contacting solid bodies.
In 1699, Amontons proposed two "laws" of friction: (1) friction force is independent of nominal (or apparent) contact area between the two bodies, and (2) friction force is directly proportional to the surface-normal component of load.
The second of these two laws gives us the equation F = μ W, where F is the friction force, W is the load and μ is the friction coefficient.
To understand this relationship, consider the inclined plane experiment shown in the figure on the left below.
As the plane is gradually tilted up and the incline angle Θ is increased, the component of the force due to the weight of the block in the direction of sliding increases.
The friction coefficient, which is the ratio of the friction force F to the normal force W, is simply equal to tan Θ.
Also, the magnitude of the friction before sliding begins is always greater than that during sliding.
This difference is illustrated in the figure on the right below, where the pre-sliding friction is called the static friction and the friction after sliding begins is called the kinetic friction.
|
Wear
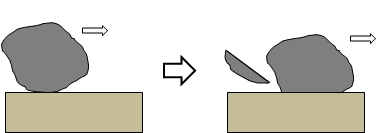
≪ More about wear ≫
The two most common types of wear are abrasive, in which a harder material removes material from a softer one, and adhesive, in which two bodies adhere to one another locally, so that material is transferred from one to the other.
These two wear modes are illustrated below.
In adhesive wear, the two sliding surfaces, or features on those surfaces, called asperties, temporarily form junctions between the two materials.
Then, as sliding continues, those junctions have to be broken.
If a junction is weak enough, shear will occur at the original interface between the two bodies and there will be negligible wear.
However, if a junction is stronger than one of the two materials, then shear may occur within the material, resulting in adhesive wear.
Abrasive wear occurs when a harder material ploughs into a softer surface, removing material from it.
This mode of wear tends to be more severe than adhesive wear in most cases.
In abrasive wear, if ploughing is done by an asperity on the harder material, the process is called two-body wear; if ploughing occurs through contact with a wear particle or debris, then the process is called three-body wear.
In addition to adhesion and abrasion, another common wear mode in mechanical components is surface fatigue. Fatigue is, as the name implies, a process that occurs after many sliding cycles. It is common in components that have rolling elements where subsurface stresses lead to cracks within the material. These cracks grow towards the surface over time, eventually resulting in worn material, a process known as pitting. Other modes of wear that can occur in some components and under some conditions are impact by erosion or percussion, chemical wear (such as corrosion), and electrical-arc-induced wear. |
Lubricants and Lubrication

≪ More about lubricants and lubrication ≫
The basic premise of liquid lubrication is that, although there is resistance to shear within the fluid due to its viscosity, that viscous resistance is much smaller than the frictional resistance during dry sliding.
The performance of a lubricated contact is therefore largely determined by viscosity.
Viscosity is a fluid's resistance to flow and is the ratio of shear stress to shear strain rate.
A fluid in which viscosity is a constant, i.e. there is a linear relationship between stress and strain rate, is called Newtonian.
However, in reality, many lubricants experience changes in viscosity due to temperature, pressure and shear rate during operating, and so behave as non-Newtonian fluids under some conditions.
Controlling these variations is important because viscosity plays a key role in determining lubricant film thickness.
A very small viscosity will result in a film that is too thin to prevent surface asperities from coming into contact, while a very large viscosity will result in sufficient surface separation, but may also lead to unacceptably high viscous friction.
In general, we want to use the least viscous fluid that results in complete surface separation.
Viscosity is measured using an instrument called a rheometer such as the one in our lab shown below.
|
Other Topics in Tribology
There are several topics that are integrally related to the core areas of friction, wear and lubrication, but that deserve their own description. These are surface roughness, contact mechanics and nanotribology. Each topic will be breifly introduced here.
≪ More about other topics ≫
The behavior of sliding interfaces can be significantly affected by the roughness of the surfaces of the two bodies.
Surface roughness is typically measured using profilometry and is often reported as the root-mean-square value of the height of the surface features, or asperities.
Other parameters that describe surface roughness are the average of the surface heights and the skewness and kurtosis of the distribution of surface heights.
The effect of roughness on friction and wear is dependent on the type of sliding.
For example, larger roughness will increase friction and wear in an interface that is dominated by abrasion while it may decrease friction and wear in an adhesive interface.
Regardless, roughness is a key property of any sliding interface.
An emerging field within tribology is friction, wear and lubrication at the nanoscale, called nanotribology. Nanotribology is relevant to a variety of novel small-scale devices as well as characterization tools, all of which rely on the nanoscale contact between two materials to function. Nanotribology is also scientifically fascinating because some of the "laws" that we use to describe larger-scale tribological phenomena no longer apply at the nanoscale. A significant amount of research in this area is performed using an atomic force microscope, where an extremely sharp probe interacts with a surface. The contact between the probe and surface is only nanometers in size. Such contacts have been found to exhibit unique and sometimes counterintuitive behavior, and understanding that behavior is the focus of many exciting research projects currently underway. |